Bugs Inside of Bugs
Moran Introduces the Insect Microbiome

You’ve heard about the human microbiome. Now, get ready for the…insect microbiome?
“It’s been a revelation to realize that a lot of what we see in insect biology is due to the microbes living in them,” said Dr. Nancy Moran, an evolutionary biologist and entomologist from the University of Texas at Austin.
In a recent WALS lecture, “The World of Insect-Bacterial Symbiosis: What We Have and Have Not Learned,” Moran delivered a “broad talk for a broad field that has developed a lot.”
If this is your first time hearing about the insect microbiome, you’re not alone. Moran was among the first researchers to study insect-bacterial symbioses in the early 1990s, but progress was slow until molecular tools such as next-generation sequencing became widely available in the mid-2000s. The reason? Many of these microbes cannot grow outside of their host, so next-generation sequencing enabled researchers to isolate microbial genetic material within their insect host’s tissues.
Organisms that form the insect microbiome are called “symbionts” and can be classified into four general categories: primary, secondary and host-specialized symbionts, and environmental microbes.
Primary symbionts are essential for their hosts’ development and daily functions. They are transmitted from mother to offspring (also called vertical gene transfer) and are housed in specialized cells called bacteriocytes.
A common example is the pea aphid. Its primary symbiont is Buchnera aphidicola, which was named in honor of Paul Buchner, an embryologist who first noticed the symbionts inside aphid embryos.
Aphid prenatal development occurs inside the mother’s body, and the Buchnera symbiont colonizes the embryos very early in development. This trusty bacterium performs a vital task for its host: synthesizing essential amino acids.
Aphids consume plant phloem sap, which is not a nutritionally balanced food. Buchnera makes up for this by recycling the aphid’s metabolic waste into amino acids. Primary symbionts of various beetles, cockroaches and whiteflies also synthesize amino acids for their hosts.
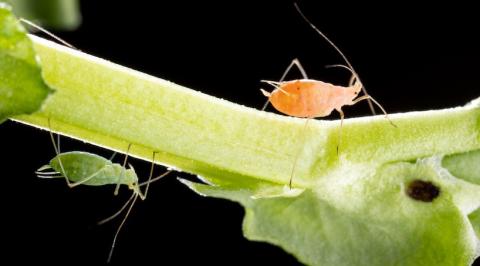
Photo: Moran Lab
Human blood is a poor source of B vitamins, so you can thank the primary symbionts of bed bugs, lice, ticks, tsetse flies and others for enabling their hosts’ vampiric lifestyles.
The evolution of primary symbionts has been millions of years in the making—more than 450 million years (when life came onto land), specifically. Moran and her collaborator Dr. Paul Baumann examined the molecular phylogenies—analyzing and comparing the genetics of species to see how they evolved over time—for numerous host-symbiont pairs.
“It turns out that the host and symbiont phylogenies map onto each other exactly,” Moran explained. Translation: the host and symbiont evolved together, and the evidence is in their genes.
One downside to this togetherness (for researchers, at least) is that primary symbionts cannot survive outside their hosts and therefore cannot be cultured in the lab. Secondary symbionts are a bit more flexible.
Pea aphids also have a secondary symbiont: Hamiltonella defensa, a pathogen-like bacteria that lives mostly in its host’s hemolymph (the insect equivalent of blood). It can be transferred maternally and also horizontally (from donor to recipient), which is similar to how infectious pathogens are transmitted.
Hamiltonella helps defend its host from a species of parasitoid wasp. Understanding the symbiont’s role is important because the wasps are a common form of aphid biocontrol. However, aphids have a “huge variation of susceptibility” to the predator.

Photo: Moran Lab
Aphids who were given the bacteria in the lab were still susceptible to the wasp—unless the bacteria contained a bacteriophage called APSE. The phage genome encodes multiple toxin genes that are useful for combating parasitoid wasps, but there is a tradeoff involved. The phage is detrimental to Hamiltonella, but the compromise still works in the bacteria’s favor if its aphid host lives in an area where the wasps are present. APSE does not persist in wasp-less environments, though, because there is no benefit to the host balancing out the cost to its symbiont.
Perhaps the perfect place for the next category of symbiont is a beehive. Host-specialized bacteria transmit through social contact. Moran’s research has focused on the honeybee gut microbiome, which she discovered is “dominated by highly host-specific bacteria.” The hindgut of a honeybee contains five core species, each adapted to its specific microenvironment.
Honeybees acquire their microbiota in their first four days of life as adults, through social contact with other hive workers. Agricultural herbicides and antibiotic usage (a common beekeeping practice) can disrupt the microbiota, causing greater in-hive mortality and greater susceptibility to pathogens.
In the lab, Moran found that microbiota-free bees die very quickly when exposed to a bee pathogen, and even a single inoculation of one microbiome species is enough to offer some protection. A full suite of gut microbes is most protective.
The final category of insect symbionts Moran discussed was environmental microbes. These bacteria may colonize their insect hosts and have an impact on their physiology, but the host does not have much of an impact on the bacteria.
One “really striking example,” Moran shared, is the gut bacteria in mosquitoes. Mosquitoes must have a population of gut microbes in order to develop into mature adults. Their microbes have not “co-evolved” with them like pea aphids and Buchnera, but are somehow still crucial to mosquitoes.
Can insect symbionts be useful to human health? Wolbachia is a common symbiont in many insect species. It can function as a primary or secondary symbiont, and researchers in the World Mosquito Program have discovered a way to use it to control dengue virus. They transferred Wolbachia from fruit flies into lab-raised Aedes aegyptii mosquitoes and then released them into areas with ongoing dengue transmission. The Wolbachia carriers are poor vectors for dengue and quickly spread through the population as they are passed from mother to offspring, eventually eliminating dengue virus in the local region.
Symbionts “provide the potential for manipulation, as well as targets for control measures,” Moran summarized.
To truly understand insect biology and its potential to shed light on our own biology, she concluded, we must look at the bugs inside of bugs.
View the archived lecture at: https://videocast.nih.gov/watch=51171.