Platt Explores Softer Side of Bioengineering
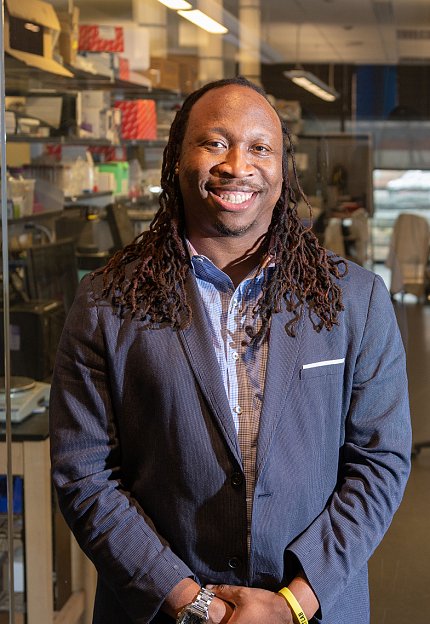
Photo: Allison Carter/Georgia Institute of technology
Dr. Manu Platt has a reputation for exuding enthusiasm when talking about his work. The biomedical engineer recently delivered an animated presentation that spanned his work on tissue remodeling, which underpins his research toward finding new therapies for sickle cell disease (SCD).
Platt arrived at NIH a year ago to launch the BETA (Biomedical Engineering and Technology Acceleration) Center, an NIH-wide data resource and community to accelerate tech-driven, interdisciplinary research.
“But the other part of my job, which was the enticing part of coming to NIH from Georgia Tech,” he said, “was I still get to run my research lab,” called MATRICES (Mechanics and Tissue Remodeling Integrating Computational and Experimental Systems).
Platt began the talk by professing his fascination with his field, which encompasses much more than the imaging techniques people usually associate with the specialty.
“There’s the softer side of biomedical engineering,” he said at the lecture, “Strokes with Sickle Cell Disease: Dynamic Interplay Between Biomechanical and Biochemical Stimuli,” held in the Porter Neuroscience Center. “It’s using math and physics to solve health-related problems.”
Platt’s lab studies the degradation of proteins, which can lead to clogged arteries and other forms of degeneration and disease.
There are many kinds of enzymes that degrade other proteins, he explained. His lab focuses on cathepsins—the most potent collagenases—which break down collagen in damaged tissue. Inhibiting cathepsins could potentially stimulate healthy tissue growth.
Pharmaceutical companies have tried developing cathepsin inhibitors; 16 of them were tested in human clinical trials.
“Sadly, zero made it through FDA approval and into our medicine cabinets,” Platt said. “Many of these drugs failed not because they weren’t efficacious…but due to [serious] side effects.”
The failure to date of approved cathepsin inhibitors led Platt’s lab to try to quantify the amount of active cathepsins, which is critical for proper dosing.
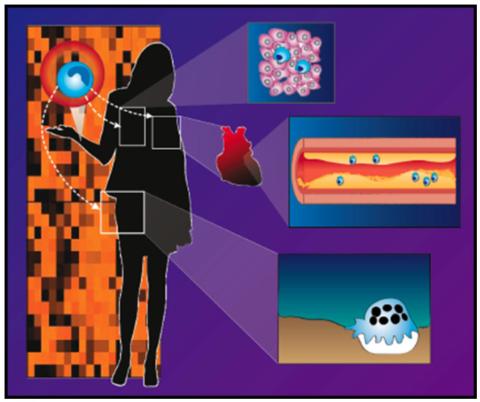
Photo: MATRICES/NIBIB
They turned to zymography—an inexpensive activity method—to look at different cathepsins implicated in tissue-destructive diseases. His lab developed a computational kinetic model enabling them to detect the amount of active protease in multiple types of cathepsins at once. This was a strategy that traditional biochemists had not pursued previously.
“The biochemists [developing the inhibitors] were looking at one enzyme and one substrate and we know the cells release more than one at a time, and we were concerned about side effects,” he said.
From their experiments, Platt’s team identified a process called cathepsin cannibalism, in which one cathepsin degrades another instead of just degrading its target. The finding changed their algorithm. “Now we had an actual mechanism,” he said, which may improve drug research.
“I love mathematical modeling,” Platt said. “When it became clear you could save on experiments, [by first] figuring out what things were not at all possible, I said, ‘Sign me up!’”
Additional experiments are underway to understand and correct what’s behind the inhibitor’s off-target effects.
Platt is also applying this research to help find new solutions to SCD, an excruciatingly painful, inherited blood disorder caused by a mutation in hemoglobin—the iron-rich protein in red blood cells that delivers oxygen throughout the body.
In SCD, red blood cells are extra sticky. They accumulate, become sickled in the capillaries, get stuck and block blood flow.
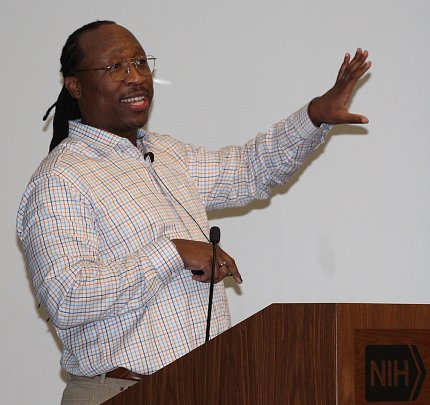
Photo: ray macdougall/NIBIB
SCD puts patients at high risk for stroke and is the biggest cause of childhood stroke. Children at greatest stroke risk need a monthly blood transfusion. Platt’s lab is looking at the biomechanics of blood flow patterns to help find alternative treatments.
The cerebral vasculature is bendy and twisty, Platt explained. At sharp turns, blood flow changes and puts blood vessels at risk for remodeling. Cathepsins are turned down or off under unidirectional blood flow, he said.
“It’s a great protective mechanism…so blood flow stays open as long as possible before plaque overgrows,” he said. But under sites of disturbed flow, cathepsins turn up to remodel the artery that leads to stroke. Could inhibitors block the path to prevent cathepsins from degrading collagen and elastin in the artery wall?
Using MRA (magnetic resonance angiography) to reconstruct the cerebral arteries of children, Platt saw that inflammation led to increased proteases that would remodel the artery wall. They also found a signaling pathway that turned on a specific cathepsin downstream of sickle cell. If they had a JNK (pathway) inhibitor, they could reduce that cathepsin signal. Now, they have a drug target.
About 11% of children with SCD have a major stroke by age 16. Children with SCD between ages 2 and 6 are at greatest risk.
“That’s the greatest risk for infarctive [silent] stroke,” he said. “Then the risk goes away but by the time patients begin to hit their early 20s, they are now at their greatest risk for hemorrhagic stroke. When we start to see their arteries become expansive and blown out, we’re seeing this aneurism—or remodeling—that could be putting those individuals at risk for hemorrhagic stroke.”
To try to understand why children are at greatest risk of arterial remodeling and stroke, Platt’s team uses a cone-and-plate apparatus in their lab to recreate blood flow profiles. They can then culture the cells over time and do biochemical analysis.
Further experiments with sickled mice injected with JNK inhibitor daily from 1-3 months old protected the elastin in the artery wall but did not produce the intended effect of preserving the mechanical properties of the artery wall.
“This is why you test hypotheses,” said Platt. They then went out to 5 months and found a JNK inhibitor protected collagen in the artery wall, protecting the vasculature from remodeling and loss of its mechanical integrity.
A few months ago, the FDA approved the first gene therapy to treat SCD.
“This is fantastic, but this would still require the patient to undergo a hematopoietic stem cell transplant (HSCT),” Platt said. “So we have been doing studies looking at, even after HSCT, [whether] the mice’s cerebral vasculatures are protected from further elastin and collagen loss, or does some of that earlier damage, once initiated, continue?”
Combining elements of biochemistry and bioengineering, Platt’s experiments continue toward understanding and reversing tissue degradation that underlies stroke risk in SCD and the progression of other diseases. His lab’s goal is to develop effective, accessible treatments that make it into the medicine cabinets of people who need them around the globe.