Potential Covid Use
Anderson Explains Role of Nanoparticles in Vaccines

If and when effective vaccines for Covid-19 are developed, some will surely rely on a nanoparticle delivery system whose origins lie in decades of painstaking groundwork.
Offering a tour of that effort on July 15 was Dr. Dan Anderson of MIT, who gave the ninth lecture in NIH’s Covid-19 scientific interest group lecture series.
The poster child for the talk was the mRNA vaccine that had just been reported on in the New England Journal of Medicine; the Moderna candidate, whose promising early results were described, relies on nanoparticle delivery.
“One of the heroes of this story is the nanoparticle,” said Anderson, professor of chemical engineering and of health sciences and technology at the MIT Koch Institute for Integrative Cancer Research.
“Encapsulating RNA in a package that can travel through the bloodstream and reach target cells is quite a challenge,” he explained. “Endocytosis is how it gets into the cell. Then it has to escape the endosome and release its payload. Decades of work have gone into this. It isn’t easy.”
Years of experiments on animal models have shown that injected nanoparticles usually end up in the organs that filter blood—liver, spleen, bone marrow and kidney.
But, as Anderson pointed out, “RNA or DNA is simply not a great drug. It does not cross cellular membranes. We need expression of these constructs to get function.”
An important precursor to nanoparticle vaccinology is basic research on small interfering RNA (siRNA), a breakthrough that won the 2006 Nobel prize. siRNAs can seek and destroy complementary strands of RNA. “In essence, we can turn off any gene we want,” Anderson said.
He described three key steps to turn nucleic acids into drugs: sequence selection, chemical modification and encapsulation.
“The first question with nanoparticles is, what do you build it out of?” Early biomaterials, such as the artificial heart, were made of material found in ladies’ girdles—polyether urethane. The tubing used for dialysis originated in sausage casing (cellulose acetate). The first vascular grafts came from the world of clothing—the synthetic fabric Dacron.
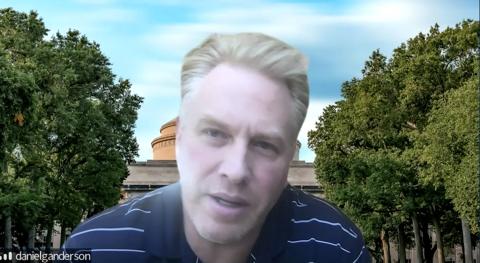
Since nothing off the shelf suggests a nanoparticle, scientists have engaged in what Anderson called the rational design of biomaterials. Biodegradable sutures, developed in the 1970s, are an example of this approach.
“How do we build the perfect material if we don’t know the design criteria?” he asked.
Anderson and his colleagues believe the best approach is to test lots of options, using four basic building blocks: a helper phospholipid, cholesterol, polyethylene glycol lipids and immune lipids.
“Every nano vaccine manufacturer is focused on figuring out the structure of ionizable lipids,” he said.
Anderson has 15 years of experience with DNA delivery systems that harken back to such early compounds as DOTMA, DOTAP, DOPE and DOGS.
“The key question is, how do we increase the diversity of these compounds?” he said. “It’s a chemistry problem. The goal is a cationic lipid.”
One early success in developing RNA therapeutics was use of siRNA to silence the TTR gene, which, when misfolded, causes transthyretin-mediated amyloidosis, a serious liver disease.
The treatment was effective in primates, which Anderson said “surprised us. It was not as hard as we thought to make lipids that could do this.”
The first siRNA lipid nanoparticle was approved for human use in August 2018, in a drug called patisiran.
“This was proof to the field that these particles…actually can be translated and approved as medicine,” Anderson noted. “This inspired us…These types of particles could have broader use.”
They decided to take advantage of endogenous lipid-trafficking pathways in the body. These pathways feature chylomicrons, which is where the fats in the last Snickers bar you ate ended up.
While liver is a relatively easy target for nanoparticles, other targets include endothelium and perhaps even immune cells, including peripheral blood leukocytes. That would enable nanotherapy for some infectious diseases, Anderson said.

His team has been able to silence 5 genes in the lung in vivo using nanoparticles, and he cited a report of 20 genes being knocked down by a single particle.
At the moment, all cells within the liver in animal models can be targeted, as can the endothelium of many organs, including kidney, liver, spleen, heart, skeletal muscle and lung. Also amenable to nanotherapy are leukocyte populations including monocytes, macrophages and dendritic cells, along with a variety of tumors, and even some T and B cells in primates.
Anderson said his MIT colleague, Nobel laureate Dr. Phillip Sharp, has labeled the new technology “modular pharmacology.”
The Moderna vaccine described in the recent NEJM article uses mRNA to activate, not silence, a gene, as with siRNA. “It’s even more challenging than siRNA,” said Anderson. “It’s much bigger.”
It is not enough, he warned, to create an mRNA that encodes an antigen to SARS-CoV-2. “You also need to activate the immune system and have the antigen present for the correct amount of time in order to get the appropriate response.”
In a mouse study, mRNA led to large amounts of circulating protein produced in the liver, as modeled with the kidney hormone EPO. DARPA scientists have been investigating mRNA therapies that can produce antibodies against infectious agents used on the battlefield.
mRNA delivery is not limited to the liver, Anderson said. “Nanoformulations can be generated to express mRNA in different tissues.”
Nanoparticle mists could be inhaled, as with a nebulizer. “We can get very high expression in lung epithelium in animals,” he reported. One company is exploring this approach for patients with cystic fibrosis.
Anderson concluded with a discussion of genome editing using the CRISPR-Cas9 system, which permanently modifies DNA for some beneficial purpose.
“Can we permanently turn genes off in vivo?” he asked. Using chemically modified guide RNA to direct such editing, Anderson thinks better versions of the system can be developed, leveraging knowledge gained in studies of antisense molecules.
Scientists are currently trying to craft a nanoparticle that can permanently lower cholesterol; one candidate resulted in a 35 percent reduction in mice.
“I invite the creative scientists at NIH to propose gene targets, to either partially or fully knock them out, in infectious diseases,” Anderson said.
Already, a single-particle Ebola vaccine has been made whose payload targets three strains of Ebola, Anderson noted. “It offered complete protection in mice from a lethal dose of the virus.”
He imagines second-generation vaccines delivered by nanoparticle that will target cancer and Covid-19, as more is learned about lipid formulations and the best pathways to target.
The full talk is available at https://videocast.nih.gov/watch=37814.