What Came First?
Biochemist Lane Talks Theory on Origin of Life
According to biochemist, writer and University College London Professor Dr. Nick Lane, “Life is a guide to its own origin.”
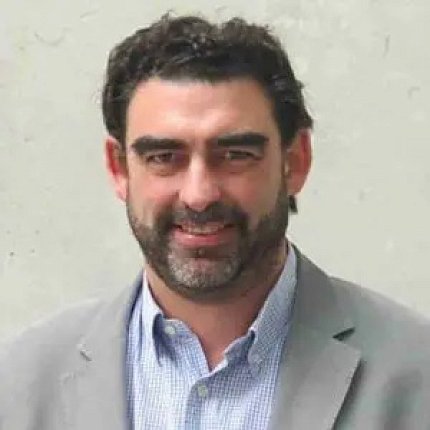
The idea seems “circular,” he admitted, but he proposed that researchers can use qualities that are common to all life on Earth (energy, metabolism and genetic information) to elucidate where it all started.
Lane spoke to a hybrid NIH audience recently in a lecture titled “On the Origin of Cells and Genes (and Why It Matters to Medicine),” sponsored by the Foundation for Advanced Education in the Sciences (FAES), the Office of Intramural Research (OIR) and Demystifying Medicine series.
“It feels presumptuous to come to NIH and talk about medicine, so I’m going to talk about the origin of life instead,” the biochemist joked.
Researchers agree that life likely originated somewhere around 3.8 billion years ago, but the specifics are lost to time.
“I’m vulnerable immediately to a charge of making it all up,” Lane acknowledged. “But it’s fun to think about.”
Traveling Back in Time
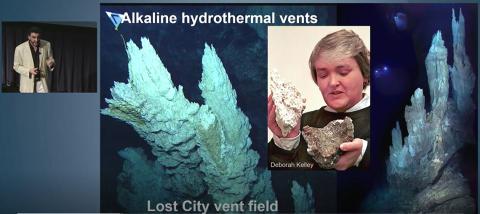
What did our planet look like almost four billion years ago? Picture a “sterile, inorganic, wet, rocky planet,” said Lane. And yet, the first single-celled organisms arose in these seemingly inhospitable conditions.
Lane studies bioenergetics, or the way energy flows through living systems. And, at the most basic cellular level, energy is generated by the Krebs cycle. This cycle also produces lipids and the precursors of certain amino acids. Lane and other researchers think this flow of energy may have helped create the first “protocells.”
But how could the Krebs cycle generate energy 3.8 billion years ago when there were not yet any organisms in existence?
All About Gradients
Alkaline hydrothermal vents were essential to this process. The porous minerals that make up these vents are alkaline (or high pH), along with the seawater inside them. In the prehistoric ocean environment, these alkaline vents opened up into acidic (low pH) ocean water outside. In an inorganic environment, a pH gradient can drive reactions between hydrogen and carbon dioxide.

The hydrogen-rich water inside the pores of the vents mixed with the carbon dioxide-rich ocean water. Additionally, the alkaline water inside the vents (about pH 11) also made the hydrogen much more reactive. The vents’ pores were also lined with catalytic compounds like iron sulfide, which created a natural proton gradient.
This gradient “effectively drove the reaction between hydrogen and carbon dioxide,” Lane explained, “lowering the [energetic] barrier to that reaction happening and driving the reaction to make Krebs cycle intermediaries and then possibly amino acids and some lipids.”
Additionally, if lipids were present, then they could have potentially made a bilayer across the openings of each of the pores, making protocells. In these partially closed systems, under the right circumstances, the protocells could form not just amino acids and lipids but also sugars, and ultimately nucleotides.
“It may sound hard to believe, but every step is provable, at least in principle,” Lane assured his audience.
Inside the Lab
The proof he discussed has been researched in numerous labs over the years. Lane’s group used microfluidic chips to mimic the flow of alkaline fluids and ocean water through a permeable barrier and observed that it did drive carbon dioxide reduction and build small amounts of organic molecules.
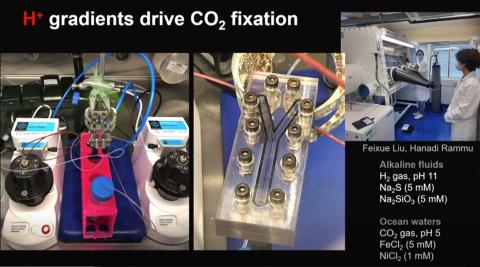
More than 20 years ago, another research group demonstrated that long-chain lipids can form under hydrothermal conditions. Lane’s group built on this research, mixing the resulting long-chain fatty acids under the original hydrothermal vent conditions to see if they could make protocells. And they could: microscopic imaging revealed “robust protocells with bilayer membranes and aqueous interiors,” Lane found.
His lab also conducted amino acid synthesis experiments, “recovering the metabolic map as we know it.” He theorized that the genetic code is structured by metabolism because the universal core of biochemistry is “older than genes [themselves].”
Bacteria and archaea—the oldest forms of single-celled organisms—both contain the exact same biochemical processes, even though the genes that encode their enzymes and catalyze reactions are not conserved in bacteria and archaea. They have “different genes encoding different proteins catalyzing the same chemistry,” Lane elaborated. “The easiest explanation for that is the chemistry came before the genes.”
Why Does It Matter to Medicine?
About 538 million years ago, oxygen levels in the atmosphere rose significantly and life on Earth diversified in an event known as the Cambrian explosion.
Prior to this, cellular life relied mostly on the reaction of hydrogen and carbon dioxide to make Krebs cycle intermediates, which is actually the reverse of the modern-day Krebs cycle. The oxidative version of this cycle was made possible by the presence of increased oxygen, allowing organisms to harvest energy more easily, grow larger and form more complex food chains.
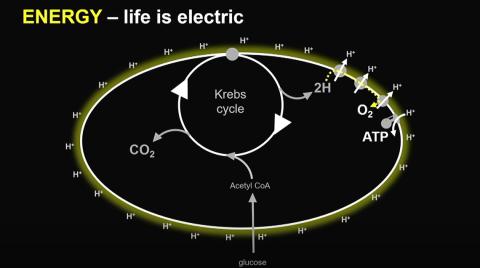
Human cells use the oxidative Krebs cycle, but damage to some of the structures involved in the cycle can cause a buildup of certain molecules.
“Essentially, the damage alters the flow of molecules in the Krebs cycle and…gives the epigenetic signal to grow,” Lane explained, which happens to be “just what cancer cells want.” This reductive Krebs cycle does not drive cancer, but creates a more permissive environment. Researchers have noted similarities in other conditions such as diabetes and Alzheimer’s.
“I’m not presenting this as right or wrong,” he cautioned, “just a way of seeing it that may give you the impulse to think about these questions.”
View the archived lecture at https://go.nih.gov/g95grxn.