‘Method of the Year’
Intramural Cryo-EM Lab Achieves Atomic Resolution

In the late 1990s, Dr. Sriram Subramaniam began to feel restless at work. After a few years of studying how fruit flies see, the then-junior faculty member at Johns Hopkins University was looking for something new to do. He found himself one day sitting across from NIH deputy director for intramural research Dr. Michael Gottesman and NIH distinguished investigator Dr. William Eaton of NIDDK.
For some time, explained Subramaniam—a biochemist at heart and by training—he had wanted to “walk inside” a retina cell (or any cell, for that matter) and be able to see all of it, inside and out. He had an idea to employ electron microscopy in this quest, he told the two veteran scientists. His interests seemed to match Eaton’s and Gottesman’s desire to bring more electron microscopy to the NIH campus. Intrigued, Eaton, a longtime structural biologist, asked whether Subramaniam had much experience with electron microscopy. Not really, Subramaniam admitted, but “nobody had told me I couldn’t do it. I thought it was a worthwhile thing to do…I had never done anything like this, but that didn’t seem to bother them.”
The time was ripe. NIH was amid its historic budget-doubling era. Funds for high-risk research were available for intrepid investigators with a solid pitch. NIH took a chance on Subramaniam. By 2000, he was on staff at NCI. A decade and a half later, results from NIH’s investment in EM are being hailed throughout the science world. Not only does Subramaniam’s lab now hold the world record for the highest resolution achieved using cryo-electron microscopy (cryo-EM), the team also holds the spots for the second- and third-highest resolution structures obtained using this technique. But that’s jumping ahead of the story.
The Early Years
Over the 10 years after Subramaniam joined NIH, he built a highly diverse team, with expertise ranging from microbiology and protein biochemistry to imaging, computer programming and electrical engineering.
“Our lab has always been full of people with myriad and eclectic interests, and spanning a wide age range,” he said. “Early work that I and Dr. Jacqueline Milne did resulted in a molecular model for the enzyme pyruvate dehydrogenase and insights into how this molecule worked in mitochondria. Then-NIH director Dr. Elias Zerhouni actually showed this data on the floor of Congress.”
Another major breakthrough came in 2008, when the lab developed powerful computational tools and a technique called cryo-electron tomography. This enabled them to publish the first 3D structure of the intact HIV envelope glycoprotein, the complex responsible for allowing the virus to invade host cells. That same year, they also used these methods to describe arrangements of membrane protein assemblies in intact bacteria.
The Better to See You
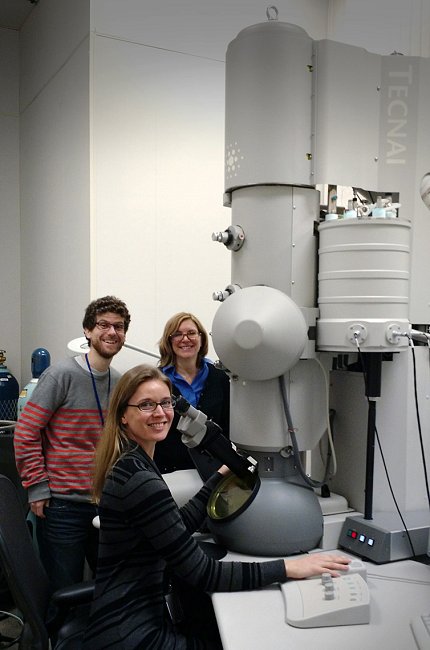
Photo: Veronica Falconieri
As they continued their forays into imaging intact HIV, influenza, Ebola and related viruses, the team also continued to sharpen efforts to see the inner details of proteins at higher resolution. A novel “Living Lab” partnership with a microscope manufacturer led to further innovations in technology and methods to achieve high resolution.
With the latest generation of microscopes, cameras and analysis tools, suddenly the protein image quality leapt forward.
In early 2015, starting with a few drops of purified protein, the Subramaniam group was the first to publish the structure of a small enzyme at atomic resolution obtained by cryo-EM—a level of detail where you can essentially make out the individual atoms in proteins. Last month, Nature Methods magazine named the cryo-EM technique “Method of the Year.” NIH director Dr. Francis Collins posted a blog about the achievement on Jan. 14.
Visualizing proteins and other molecules in the cell can be a little tricky. In the mid-20th century, scientists first started getting glimpses of the internal workings of proteins using X-ray crystallography. That technique became the go-to structural biology method. But even though the structures of a great many proteins have been studied this way, some molecules—big, wobbly or dynamic proteins or complexes that just won’t crystallize—have foiled crystallographers.
Now, cryo-EM, as the Subramaniam group and many others world-wide are beginning to show, may be ready to challenge crystallography in the 3D imaging resolution domain.
Location, Location, Location?
One important goal in advancing these methods is to find better drug targets. If, for example, you can learn how a protein involved in cancer—or any disease—is shaped, where it is situated in a cell, how it moves in its environment and what its neighboring biomaterial is like, then you can figure out the best way to alter the protein’s function and thus treat the disease.
“The interesting part is the interpretation of the structure,” Subramaniam explained. “[Protein] structure is useful in many different contexts. We like to understand how cells work. We like to understand how viruses get into cells…The structure and dynamics are generally interesting biologically across the board, but our present interest, keeping the future in mind, is to leverage this information to accelerate drug discovery.”
Staying with the architectural theme, Subramaniam compares macromolecular drug discovery to buying real estate. The protein you’re studying is the house you’re interested in purchasing. Of course you want to tour the inside, look at how the space flows and see all of the nooks and crannies invisible from outside. Just as important, however, is the neighborhood and environment around your potential residence. You want to know what else is nearby—streams and ponds, other structures—that might affect your house.
Welcome to the Neighborhood
“Very often when drugs bind, the proteins that we are targeting bind in the context of interacting with lots of other partners, so when we look at just the local target we don’t have a feeling for what’s happening,” Subramaniam said. “What’s happening with the neighbors? What’s happening downstream? We want to look at drugs bound to native proteins. Generally we want to focus on things that are hard to do by crystallographic methods, but are nevertheless important. So we want the structures of protein in as close a state as possible to what they are in the cell. Because when drugs work or don’t work, we want to know why. That’s what we’re after.”
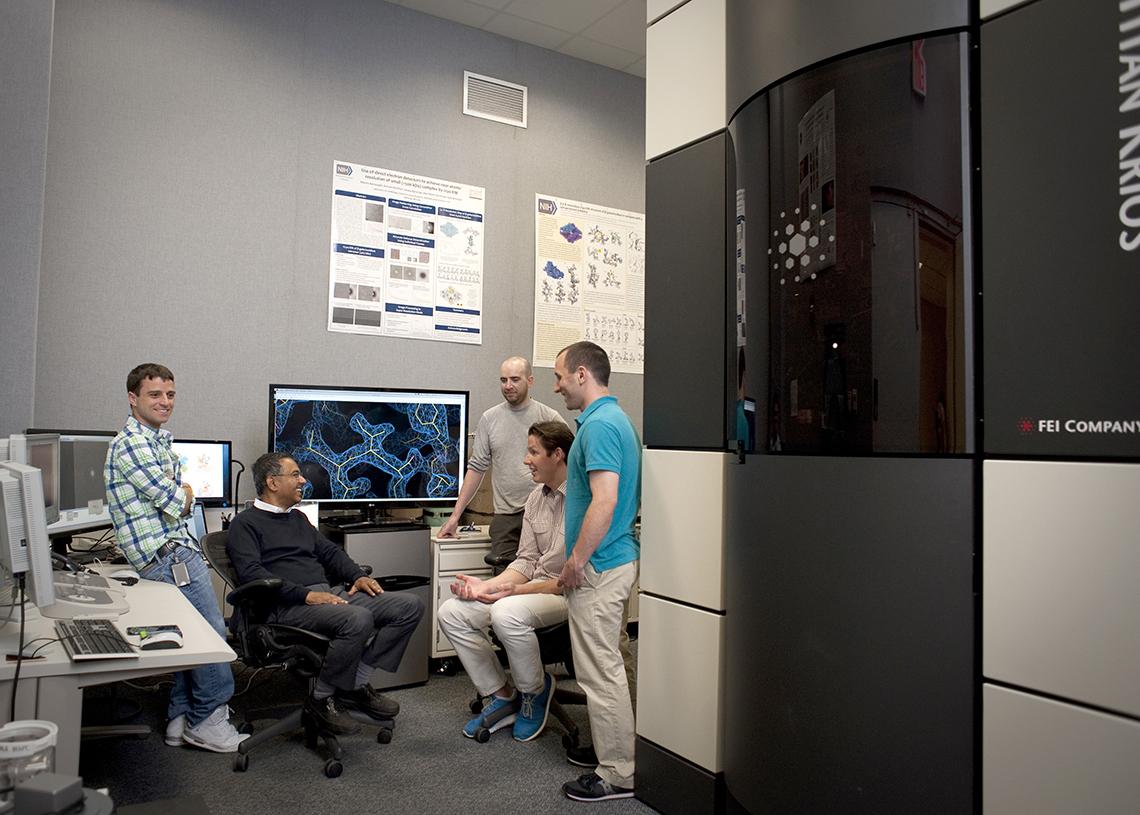
Photo: Rhoda Baer
Just this past month, the Subramaniam lab, in coordination with the NCI NExT program and several labs around the country, published the first complete structure of the cancer target protein p97 at atomic resolution. Other groups had analyzed the structure of parts of p97, but because inhibitors can bind at the junction between different sections of the protein, it was critical to image the whole protein complex.
“Being able to see in detail how this protein machine works, and how the inhibitor throws a wrench in the works, using cryo-EM—it’s a whole new world,” said Subramaniam.
So, how does his cryo-EM team decide which of the thousands of macromolecules to image?
“We cast a wide net,” he said. “Proteins important in brain function, in cancer, in viral infection, vaccines—it’s quite a broad spectrum. We’ve tried to pick important examples, where if it works, we learn something new. That’s why we picked receptors in the brain. We picked HIV. We picked cancer targets. In each case, as we push the frontier of the technology a bit more, we also at the same time try to advance a particular biological mission.”
What’s Next?
Subramaniam also intends for NIH to maintain its head start on the rest of the cryo-EM field. The goals now, he said, “are to get this method to be more broadly applicable and to accelerate the rate of drug discovery.”
Already he has the germ of a new idea forming. “I do see in the next decade a way to take on that problem [of studying drug interactions] seriously, by applying these methods,” he said. “So we can get a much more calibrated view of how drugs interact with cells. Maybe we’ll understand what works and what doesn’t work.”