Miniature Models Made to Aid Drug Development
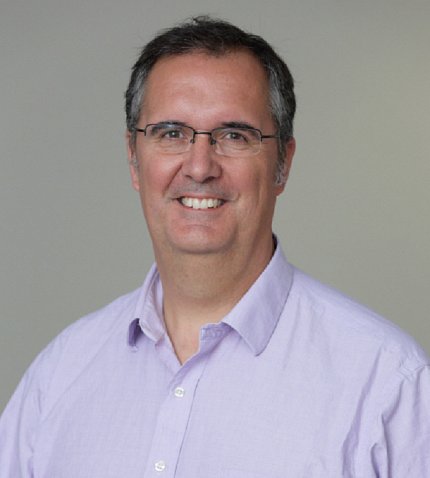
Tissue built by a 3-D bioprinter. Miniature organs grown on petri dishes or cells grown on “chips.”
Aside from sounding like medical technology from the future, these innovations all share something else: they are all microphysiological systems. And many of them are already in use at NIH.
“The naming can be confusing,” said Dr. Marc Ferrer, director of the 3-D Tissue Bioprinting Laboratory at the National Center for Advancing Translational Sciences. “Some people call these complex tissue models organoids, some people call them tissue chips.” But “microphysiological system” encompasses the whole spectrum of these miniature models.

Photo: Hesperos
According to the FDA, microphysiological systems (or MPS) are “organoid cell formations of human or animal origin in a micro-environment that provide and support biochemical/electrical/mechanical responses to model a set of specific properties that define organ or tissue function.”
MPSs are miniature tissue and organ models grown in the lab with various kind of cells from human tissues. Researchers at NCATS and elsewhere are hoping to use these technologies to solve a major problem in drug development.
“Only about 10 percent of drugs being developed [receive full FDA approval],” said Dr. Danilo Tagle, director of NCATS’s Office of Special Initiatives. “That’s...a 90 percent attrition rate.”
The high failure rate is largely due to the models researchers use to test the drugs, he revealed. 2-D cell culture systems and animal models are typically used to evaluate therapeutics, but they are not very good at predicting a drug response in a human body.
Researchers at NCATS and elsewhere are hoping MPS tech can solve this predictability gap, a big translational problem.
Toward Better Models
Very often, the effect of drugs in animals cannot be extrapolated into humans because of species differences. 2-D cell culture systems also are inadequate because they involve testing drugs on a single cell type grown as a monolayer on plastic surfaces—a far cry from being in a tissue- or organ-like environment, with its many different cell types that influence drug responses in a real human. Up until recently, though, these were the best options researchers had.
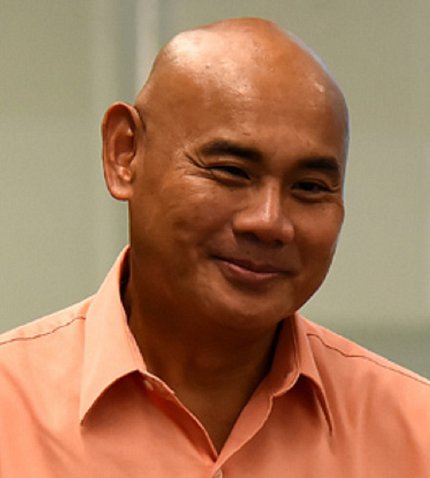
“We needed a better predictive model,” Tagle realized.
Enter MPSs.
Founded in 2011, NCATS began developing tissue chip technology in 2014. The 3-D bioprinting lab followed in 2018. Organoids and spheroids—the other types of MPSs—pre-date NCATS, but are also being studied by the center.
Making an MPS begins with human cells. Researchers have learned how to convince primary cells—those harvested directly from living tissue or organs—to grow and differentiate into models in the lab.
“We use primary cells when we can,” said Ferrer, noting that they are useful in tissue models like skin, where cells are relatively easy to get. In situations where primary cells are not easily obtained, such as the brain, researchers use adult stem cells called induced pluripotent stem cells, or iPSCs.
iPSC cells are typically derived from skin or blood samples and researchers have established guidelines for inducing these cells to become stem cell-like. Like embryonic stem cells, iPSCs are generic cells that can differentiate into any cell type, but iPSCs have the benefit of being sourced from adult tissue.
Spheroids are typically made starting from primary cells, or from iPSC cells that have already been differentiated. Organoids, on the other hand, often start as undifferentiated iPSCs that are then instructed to develop into a certain cell type, said Ferrer.
Challenge—Mimicking Organ Function

Photo: Byron Mui, Min Jae Song, Pam Robey, Marc Ferrer
Both spheroids and organoids are 3-dimensional clusters of cells; the difference comes from how the cells organize and function within the cluster.
When scientists make an organoid, they bathe the iPSCs in a fluid containing a mixture of growth factors and other compounds that encourage the cells to differentiate into the desired cell types. These cells then “self-organize” into a cellular-level model of an organ, a process that might take many weeks and even months.
Spheroids, because they are made from mature primary cells, can assemble without differentiating. They’re a “faster way to create 3-D organotypic models,” Ferrer said. Spheroids are less complex than organoids, but are still useful because they have multiple cell types functioning together. Both spheroids and organoids are useful for large-scale drug testing, particularly spheroids because they are easier to make.
Both spheroids and organoids can be used to test drug efficacy, but both also have drawbacks. There is no way to mimic the blood flow that those cells would be exposed to in a real organ, for example. And, because cells in organoids differentiate and self-assemble, it is sometimes difficult for scientists to include all the cell types needed and at the right proportions to mimic an organ.
That is where tissue chips come in. Approximately the size of a USB drive for a single organ system, these technologies are designed to mimic the structure and function of a human organ.
“Organoids and spheroids work well for modeling many tissues and organs, including tumors, colon, liver and brain,” Ferrer said, but “tissue chips are ideal when you need to mimic blood flow and reproduce ‘cross-talk’ between organs.” Researchers can even incorporate multiple organ systems onto a single, playing card-sized chip.
Chips are “probably the most complex and sophisticated way of structuring and copying organ function in [the lab],” Tagle agreed.
Chip Creation
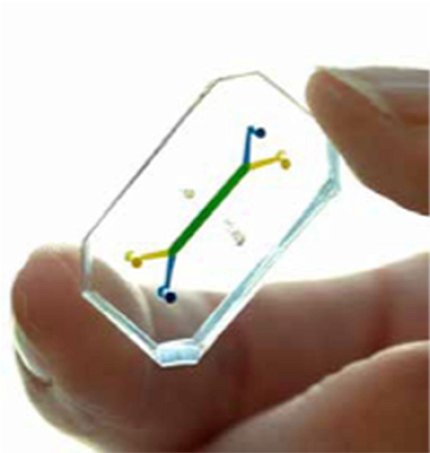
Photo: Emulate
Making a tissue chip begins with a flexible plastic, which is cut into the desired shape. Then, chambers and channels are added—either by etching or laser. The chambers are lined with differentiated iPSCs, and fluids are run through the channels to mimic functions like nutrient or blood flow. Researchers can also add sensors to measure processes such as pH or oxygenation. The sensors can even simulate mechanical stress.
A lung-on-a-chip, for example, will also have an air-liquid interface, where cells are exposed to air—just like they would be in a real lung.
“It captures the real microenvironment of the tissues” that you’re trying to model, Tagle explained.
‘Ketchup and Mustard’
The final category of MPS is 3-D bioprinted tissue.
Bioprinting employs similar techniques to traditional 3-D printing (but uses biological materials including live cells) to create models that mimic natural tissues. Researchers design the architecture of the tissue in a computer program. Then the model is printed layer by layer using primary or iPSC-derived cells mixed with “bio-ink.”
Ferrer said the process looks “a bit like mixing ketchup and mustard.” It is a labor-intensive and technically difficult process compared to making organoids and spheroids, but has the benefit of producing a much more precise model.
Future Uses
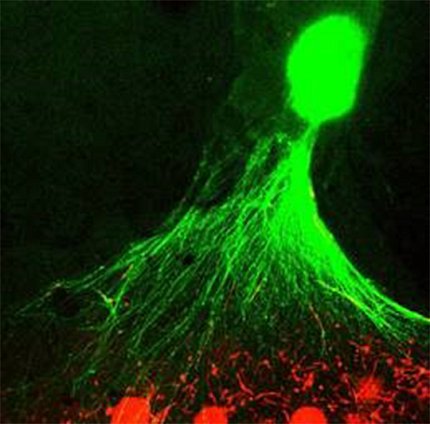
Photo: Cristina Antich Acedo, Shayne Frebert, Jennifer Colon Mercado, Min Jae Song, Michael Ward, Marc Ferrer
So, what lies ahead for MPSs? NCATS investigators say these devices will vastly improve the accuracy of pre-clinical trial drug screening.
Ferrer envisions using a combination of tissue chips, organoids, spheroids and bioprinted tissue models to aid the process of drug discovery and development. Initially, researchers could weed out ineffective drugs by testing them in a multi-well plate, each well containing a spheroid, organoid or bioprinted tissue, and one compound added to each to test its effect.
After the ineffective drugs are removed, the most promising compounds could undergo additional testing in more sophisticated and complicated tissue chip models.
MPSs also have the potential to complement animal research in drug testing.
“Animal models of disease cannot always capture the entire pathology of human disease,” Tagle explained. He cited the Alzheimer’s mouse model, which has been around for several decades but has not yielded any effective treatments.
MPSs, because they use human cells, are producing much more clinically predictive human models. MPSs are complementing animal research in drug testing. We are not yet at a point where MPSs can replace animal research entirely, he said, but “[they] can easily fill in areas where we can refine our use of animals.”
Next Steps
What will make these more practical to use?
Researchers need to account for diversity in human populations when building MPSs, because different groups may respond differently to certain diseases or treatments.
Another limitation is that researchers have not yet managed to build a model that can replicate a fully functioning adult immune system. And, investigators need to produce consistent results—so, every liver tissue chip in a trial will have the same response to the same drug.
“The challenge is getting [MPSs] out of specialized labs and into the hands of people doing basic, translational and clinical research,” said Ferrer.
At NIH, that is already in motion. NCATS is collaborating with several ICs—NEI, NHLBI, NCI, NINDS, NIAID and others—to incorporate MPSs into their research, as well as with other federal entities such as NASA, VA and FDA. Private companies are also working on their own MPSs.
MPSs “are already making a big impact,” Tagle concluded.